Introduction
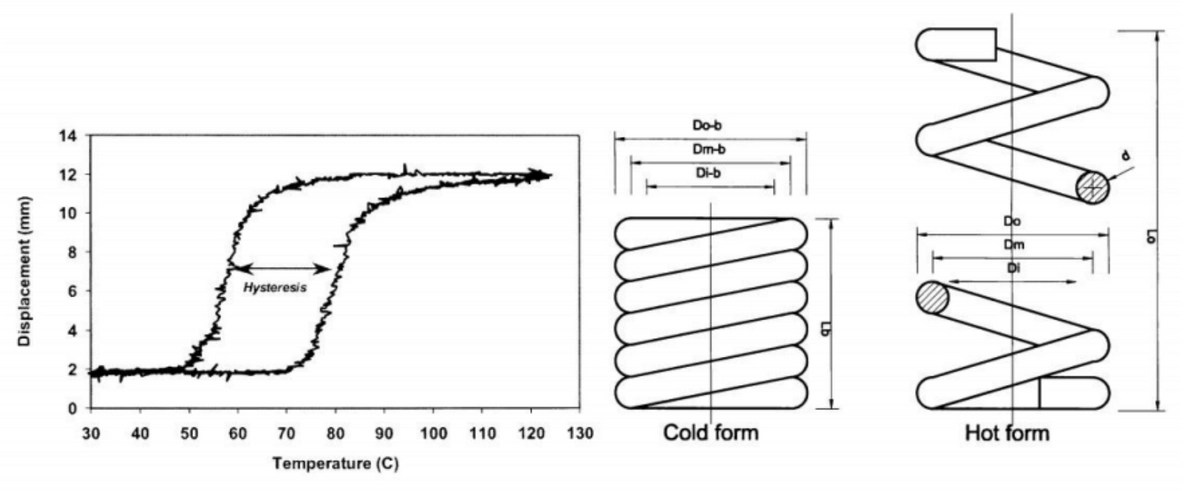
Nitinol transition temperature is a fascinating and crucial aspect of shape memory alloys. Understanding this temperature is essential for leveraging the unique properties of Nitinol in various applications. Exploring Nitinol's AF temperature and demystifying its transition temperature will shed light on the incredible potential of this material.
Understanding Nitinol Transition Temperature
Nitinol transition temperature refers to the critical point at which this shape memory alloy undergoes a phase change, allowing it to remember and recover its original shape when subjected to specific conditions. This remarkable property makes Nitinol an ideal material for medical devices, aerospace components, and other innovative technologies.
Exploring Nitinol's AF Temperature
The AF (Austenite Finish) temperature of Nitinol is the specific point at which it fully transitions from its martensitic phase to its austenitic phase. This transition plays a vital role in shaping the material's behavior and performance in different applications, making it a key parameter to consider in engineering and design.
Demystifying Nitinol Transition Temperature
While Nitinol's transition temperature may seem like a complex concept, demystifying it can lead to groundbreaking advancements in material science and engineering. By unraveling the intricacies of this temperature, researchers and innovators can optimize the performance of Nitinol-based products for enhanced functionality and reliability.
What is Nitinol Transition Temperature?
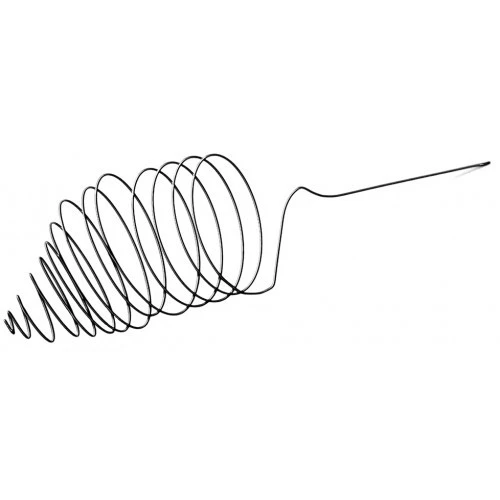
Nitinol transition temperature refers to the temperature at which Nitinol undergoes a phase transition from austenite to martensite or vice versa, leading to its unique shape memory and superelastic properties. This remarkable material is a nickel-titanium alloy known for its shape memory effect and superelasticity, making it ideal for various medical, aerospace, and automotive applications.
Definition and Properties of Nitinol
Nitinol, also known as NiTi or nickel-titanium, is a shape memory alloy with the ability to return to its original shape after deformation when exposed to its transition temperature. This remarkable property makes it suitable for applications such as stents in medical devices, actuators in robotics, and flexible eyeglass frames. Its unique combination of high strength, biocompatibility, and corrosion resistance further enhances its appeal in diverse industries.
Factors Affecting Nitinol Transition Temperature
The transition temperature of Nitinol is influenced by several factors including the ratio of nickel to titanium in the alloy, heat treatment processes, and mechanical deformation. The transformation temperature can be tailored by adjusting these factors during the manufacturing process to suit specific application requirements. Understanding these influencing factors is crucial for optimizing the performance of Nitinol-based devices.
Applications of Nitinol Transition Temperature
The ability to precisely control the transition temperature of Nitinol has led to its widespread use in medical devices such as stents, guidewires, and orthodontic wires due to their ability to adapt to body temperatures. In addition, Nitinol's unique properties have found applications in aerospace components like actuators and adaptive wing structures that respond dynamically to changing environmental conditions.
Now that we have explored the definition and properties of Nitinol as well as the factors affecting its transition temperature along with diverse applications where it can be utilized effectively let's delve deeper into what temperature is needed for Nitinol to set?
What Temperature is Needed for Nitinol to Set?
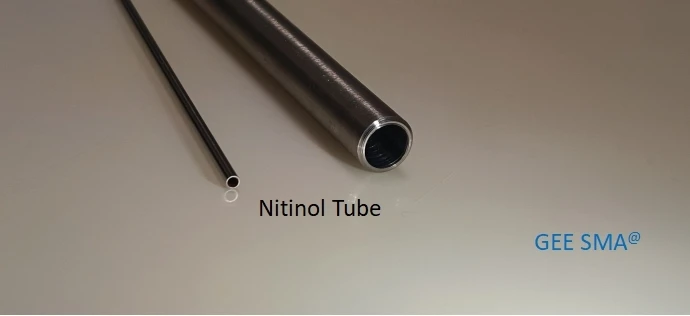
Nitinol setting temperature, also known as the critical transformation temperature, refers to the specific temperature at which Nitinol undergoes a phase change from austenite to martensite. This critical temperature is crucial in determining the shape memory effect and superelasticity of Nitinol alloys.
Understanding the Critical Transformation Temperature
The critical transformation temperature is influenced by factors such as alloy composition, heat treatment, and mechanical processing. It is essential to carefully control and monitor this temperature to ensure that Nitinol exhibits the desired shape memory and superelastic properties.
Effects of Composition on Nitinol Transition Temperature
The composition of Nitinol, particularly the ratio of nickel to titanium, plays a significant role in determining its transition temperature. Higher nickel content lowers the transition temperature, while higher titanium content raises it. Understanding these effects is crucial in tailoring Nitinol alloys for specific applications.
Common Misconceptions about Nitinol Setting Temperature
One common misconception about Nitinol setting temperature is that it remains constant for all types of NiTi alloys. In reality, different compositions and processing methods can lead to variations in this critical temperature, making it essential to customize alloy formulations based on specific performance requirements.
What is the AF Temperature of Nitinol?
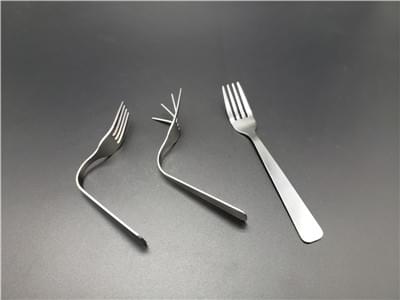
Nitinol, a shape memory alloy, exhibits a unique behavior known as the Austenite Finish (AF) temperature. This is the temperature at which the material completes its transformation from martensite to austenite upon heating. The AF temperature is a crucial parameter in determining the functional range of Nitinol-based devices and plays a vital role in various applications.
Exploring Austenite Finish Temperature
The Austenite Finish temperature of Nitinol is the point at which the material fully reverts to its high-temperature crystal structure, allowing it to resume its original shape. This phenomenon makes it an ideal candidate for applications requiring precise and controlled movements, such as medical stents and orthodontic wires.
Importance of AF Temperature in Nitinol Applications
The AF temperature directly influences the operational window of Nitinol devices, impacting their performance and reliability. Understanding and optimizing this parameter is essential for ensuring that Nitinol-based products function as intended across a wide range of environmental conditions and usage scenarios.
Controlling AF Temperature for Nitinol Shape Memory Alloys
Engineers and researchers are constantly developing methods to control and tailor the AF temperature of Nitinol alloys to suit specific application requirements. By adjusting factors such as composition, processing techniques, and heat treatment parameters, they can fine-tune this critical property to enhance the functionality of shape memory alloys.
Remember that understanding the behavior of Nitinol at different temperatures is essential for harnessing its unique properties effectively. By exploring and manipulating parameters such as transition temperatures, including AF temperature, we can unlock new possibilities for innovative applications in various industries while ensuring optimal performance and reliability.
What is Transition Temperature in Dbtt?
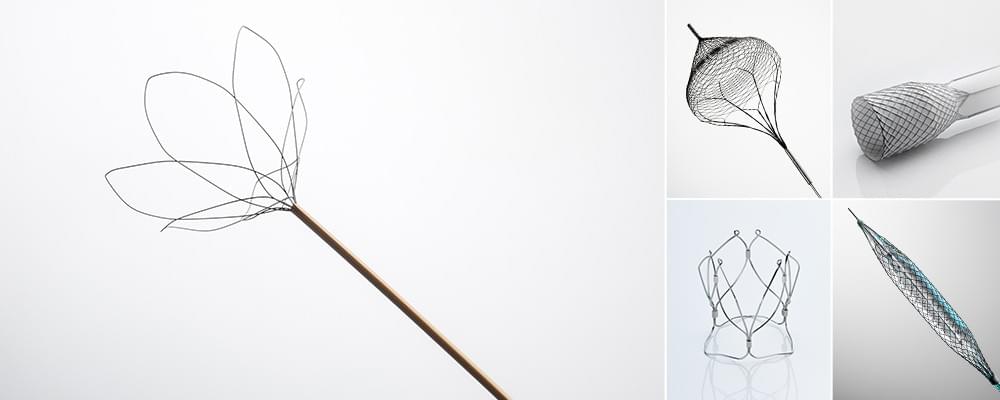
Understanding Debye Temperature and Its Relation to Nitinol
Debye temperature, also known as the Debye frequency, is a measure of the characteristic vibrational energy of a solid material. In the case of Nitinol, this temperature determines the point at which thermal energy overcomes atomic bonding forces, leading to phase transformations. Understanding this concept is crucial for optimizing Nitinol transition temperature.
Debye temperature is a critical factor in the design and application of Nitinol-based devices. By understanding this characteristic vibrational energy, engineers and researchers can tailor the material's properties to suit specific requirements. For example, a higher Debye temperature may be desirable for applications where Nitinol needs to withstand greater thermal stress, while a lower temperature might be more suitable for medical devices that require precise activation at body temperature.
Effects of Different Transformations on Nitinol Transition Temperature
Different transformations such as martensitic and austenitic have varying effects on Nitinol transition temperature. These transformations are influenced by factors like stress, strain, and temperature variations. By studying these effects, researchers can better predict and control the behavior of Nitinol in different applications.
Studying the effects of different transformations on Nitinol transition temperature is crucial for understanding how this shape memory alloy behaves under various conditions. For example, the martensitic transformation occurs when Nitinol is cooled below its transition temperature, causing a change in crystal structure. On the other hand, the austenitic transformation takes place when Nitinol is heated above its transition temperature, reverting back to its original crystal structure. These transformations can be influenced by external factors such as stress and strain, as well as variations in temperature, making it essential to thoroughly investigate their impact.
Applications of Dbtt in Nitinol Research and Development
The concept of Debye temperature has wide-ranging implications for Nitinol research and development. It allows scientists to tailor the material's properties for specific applications such as medical devices, aerospace components, and actuators. By leveraging Dbtt, engineers can create innovative solutions that harness the full potential of Nitinol shape memory alloys.
What Temperature Does Nitinol Recrystallize At?
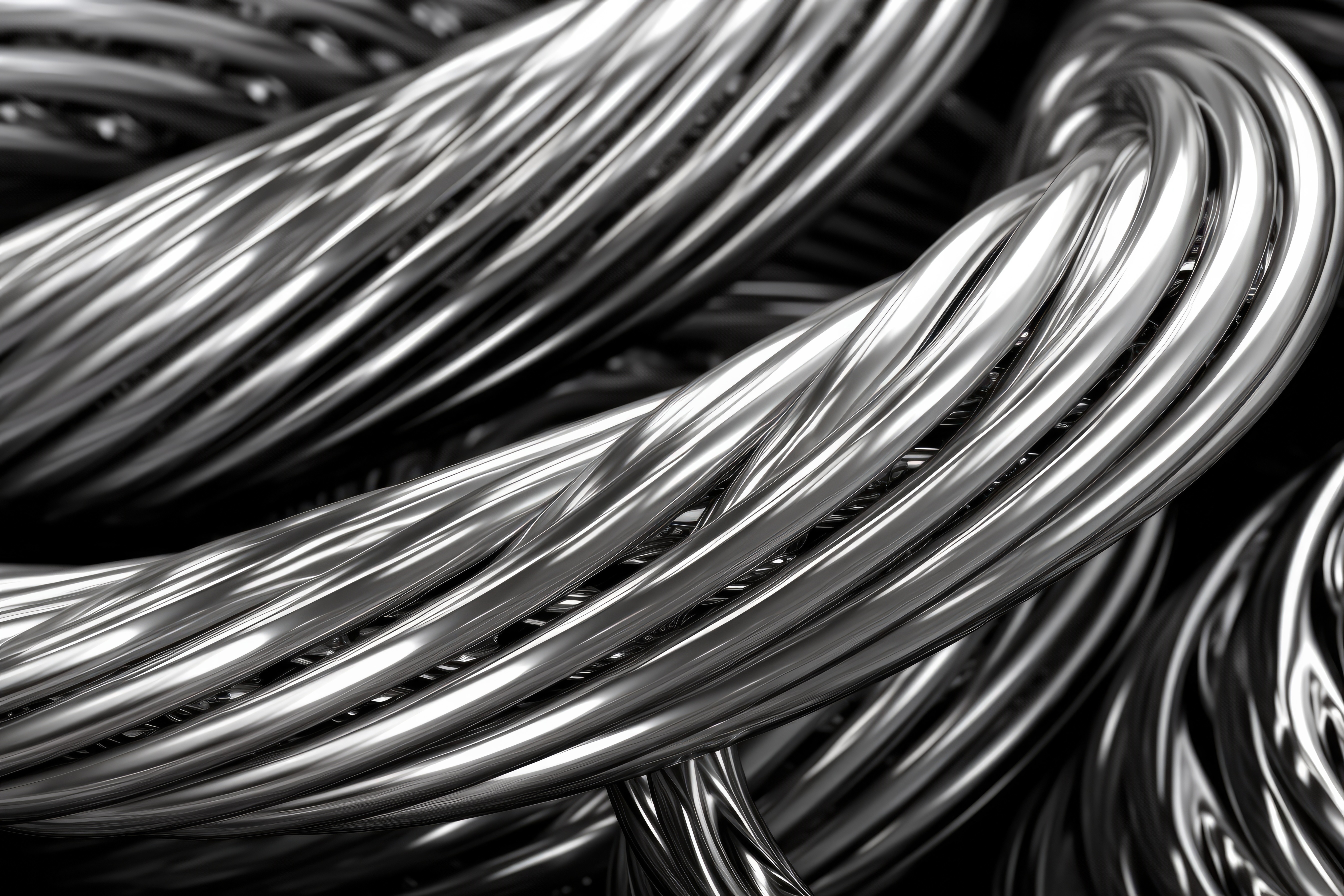
Process of Recrystallization in Nitinol
Recrystallization temperature plays a crucial role in determining the final properties of Nitinol. Lower recrystallization temperatures may not fully reorganize the grains, leading to residual stresses and reduced ductility. On the other hand, higher temperatures can result in grain growth, which may compromise the material's mechanical properties. Therefore, finding the optimal recrystallization temperature is essential for achieving the desired balance between ductility and strength in Nitinol.
Impact of Recrystallization Temperature on Material Properties
The recrystallization temperature has a significant impact on the mechanical properties of Nitinol. Lower recrystallization temperatures can result in a softer material with improved formability, while higher temperatures may lead to increased strength and hardness. Understanding and controlling this temperature is crucial for tailoring Nitinol to specific requirements.
Lowering the recrystallization temperature of Nitinol can also result in improved fatigue resistance, making it an ideal material for applications that require repetitive bending or flexing. This can be particularly beneficial in medical devices such as stents and guidewires, where the material needs to withstand constant movement without experiencing failure. By carefully controlling the recrystallization temperature, manufacturers can ensure that Nitinol components have the necessary durability to meet these demanding requirements.
Techniques to Control Recrystallization Temperature in Nitinol
Several techniques can be employed to control the recrystallization temperature in Nitinol, such as alloy composition adjustments, heat treatment processes, and precise control of heating and cooling rates during manufacturing. These methods enable engineers to fine-tune the material's properties according to the desired application, ensuring optimal performance.
In addition to the techniques mentioned above, another method for controlling the recrystallization temperature in Nitinol is through the application of strain. By introducing controlled levels of strain during manufacturing processes, engineers can manipulate the material's microstructure and alter its recrystallization behavior. This allows for further customization of Nitinol's properties, providing a wider range of options for specific applications and performance requirements.
Now that we've explored the intricacies of Nitinol recrystallization, it's evident that this process plays a crucial role in shaping the material's properties for diverse applications. By understanding how recrystallization occurs, its impact on material properties, and techniques for controlling it, engineers can harness the full potential of Nitinol for innovative solutions.
Conclusion
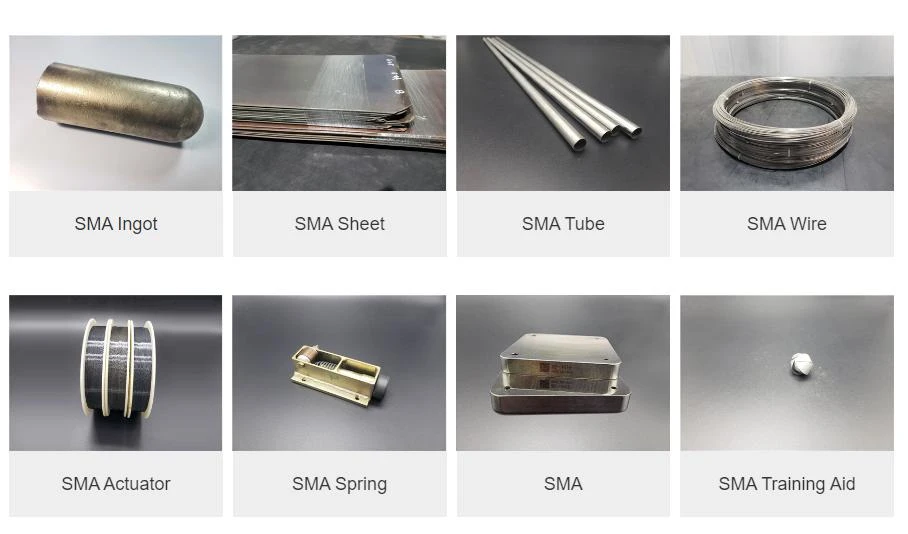
In conclusion, understanding and optimizing the nitinol transition temperature is crucial for achieving better performance in various applications. By carefully controlling the setting temperature, AF temperature, transition temperature in Dbtt, and recrystallization temperature, engineers and researchers can enhance the properties of nitinol shape memory alloys. Leveraging these temperatures opens up opportunities for innovative applications across industries.
Optimizing Nitinol Transition Temperature for Better Performance
By fine-tuning the nitinol transition temperature, engineers can tailor the material to meet specific application requirements, such as medical stents that need to expand at body temperature or actuators that must operate in extreme temperatures. This level of customization ensures that nitinol-based devices and components perform reliably and consistently, even under challenging conditions. With a deep understanding of the relationship between transition temperature and alloy composition, engineers can push the boundaries of nitinol's capabilities and explore new possibilities for its use in cutting-edge technologies.
Enhancing Nitinol Shape Memory Alloys with GEE SMA
One way to enhance nitinol shape memory alloys is by using GEE SMA (Grain Boundary Engineering Shape Memory Alloys), which involves manipulating grain boundaries to improve mechanical properties at specific temperatures. This innovative approach offers a new avenue for tailoring nitinol transition temperature to meet specific application requirements.
Additionally, GEE SMA can also improve the fatigue resistance of nitinol shape memory alloys, making them more durable and long-lasting in high-cycle applications. By manipulating the grain boundaries, the material's ability to withstand repeated deformations is significantly enhanced, opening up new possibilities for innovative designs in industries such as aerospace and medical devices. This breakthrough in material engineering allows for the development of nitinol components that can endure rigorous use without compromising performance.
Leveraging Nitinol Transition Temperature in Innovative Applications
The ability to leverage nitinol transition temperature opens up exciting possibilities in innovative applications such as adaptive eyeglass frames, self-deploying stents, and smart actuators. By harnessing the unique properties of nitinol at different temperatures, engineers can create cutting-edge solutions that respond dynamically to changing conditions.
Furthermore, nitinol's ability to return to its original shape after being deformed makes it ideal for applications in the medical field, such as minimally invasive surgical tools and orthodontic wires. This unique property allows for the creation of devices that can navigate through narrow pathways within the body and then spring back into their intended form once they reach their destination. As a result, nitinol has revolutionized the way certain medical procedures are performed, leading to improved patient outcomes and recovery times.